Could integrating ecosystem service frameworks into energy-climate modelling give an edge to low energy demand pathways? Dan Alberga investigates.
Current approaches to energy systems planning sideline the importance of biodiversity, though this trend may be changing as natural capital accounting and ecosystem service valuation efforts become mainstreamed. Could a more thorough integration of ecosystem service frameworks into energy-climate modelling give an edge to low energy demand pathways over conventional energy pathways? Or are the potential ecological outcomes more nuanced than this?
Ecosystem services & energy-climate modelling and policy
Last June, the first ever collaborative research workshop was held between the Intergovernmental Panel on Climate Change and the Intergovernmental Science-Policy Platform on Biodiversity and Ecosystem Services. The resulting 250-plus page report begins with a sobering preface summarising the risks of seeking to address climate change without considering biodiversity, or vice versa:
“The research community dedicated to investigating the climate system is somewhat, but not completely, distinct from that which studies biodiversity… This functional separation creates a risk of incompletely identifying, understanding and dealing with the connections between the two. In the worst case it may lead to taking actions that inadvertently prevent the solution of one or the other, or both issues.”
For energy policy analysts and modellers, this statement acts as a call to ramp up interdisciplinary efforts and collaboration with ecologists, conservationists, and economists seeking to restore or protect ecosystems, measure the quantity and value of natural capital stocks, and monitor changes in ecosystem services.
The current biodiversity crisis is deeply intertwined with climate change, but is not exclusively caused by it. For most species, habitat degradation and direct exploitation eclipse climate change as major threats. Why should we care about preserving biodiversity? A biodiverse ecosystem is analogous to a diversified financial portfolio; it is a necessity for ensuring resilience against external shocks. Ecosystem services are the benefits that humans derive from nature – the financial equivalent of the return generated from a portfolio of assets. Therefore, from a human perspective, what matters most is not necessarily whether a certain species exists, but how important that species is to the overall functioning of the ecosystem to provide the services that humans rely on.
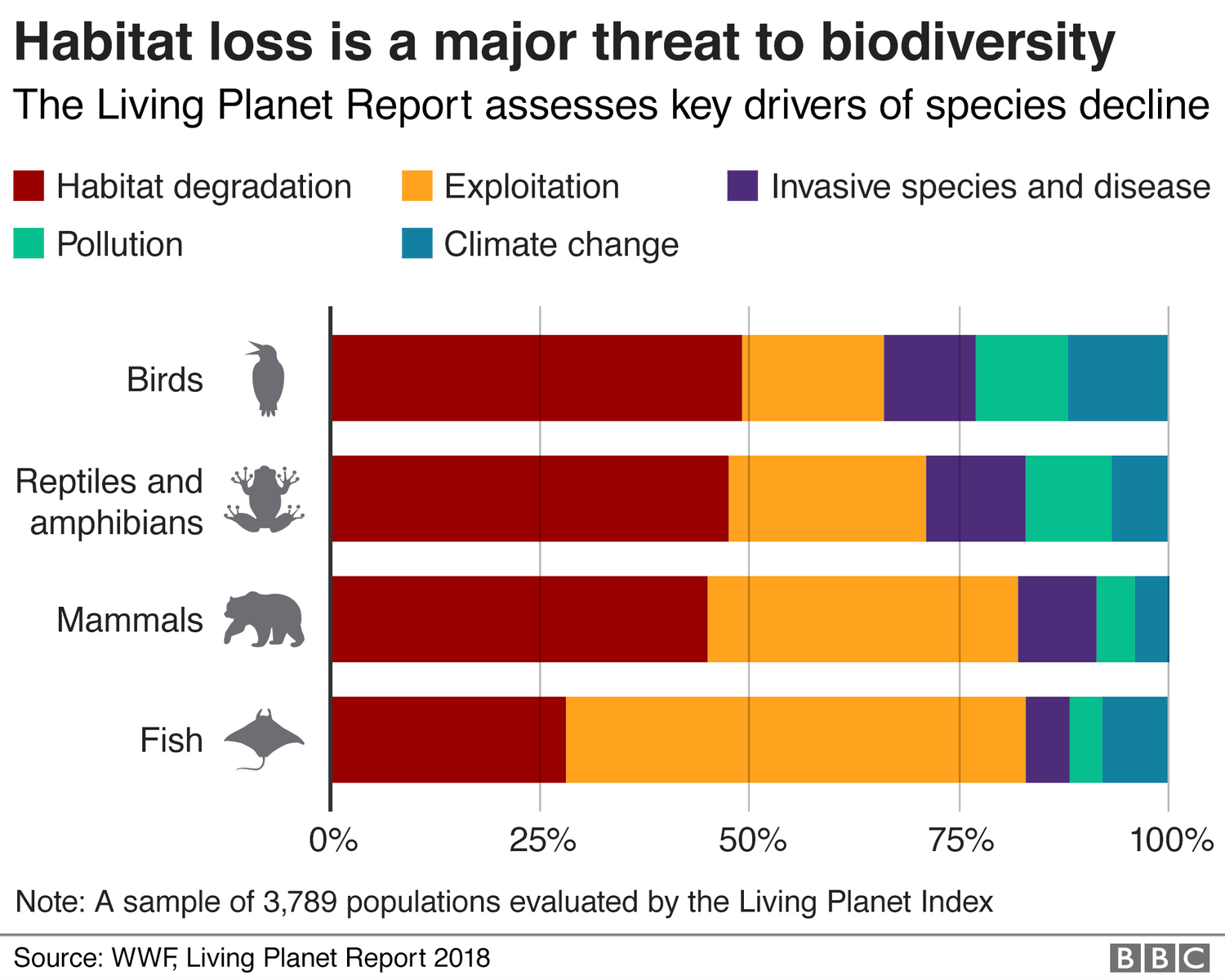
In public discourse, climate change is often presented as a more urgent threat to humanity than biodiversity loss, contradicting recent scholarship on planetary boundary studies that has sounded the alarm on the state of global biosphere integrity. However, arguing about which of the interrelated ecological crises is more urgent is a fruitless task – what is necessary from environmental managers and policy-makers is to ensure that a sound understanding of ecology and earth-systems sciences is present in all approaches. This means moving beyond GHG mitigation as the ‘holy grail’ of environmental indicators, towards the inclusion of multiple ecological measures that can accurately gauge broader ecosystem service benefits. In other words, we need to aim for ‘Net Zero Plus’ as opposed to Net Zero alone. To what extent is this approach being followed in the energy modelling community?
In their 2016 paper titled Bridging the gap between energy and the environment, Robert Holland and co-authors sought to explore this question by analysing how climate, biodiversity, and ecosystem service objectives are currently aligned. They noted that although certain biophysical indicators like acidification and land use are commonly used in energy pathways, these are problematic because “they do not capture the spatial context within which the impacts are occurring, or the ability of the ecological system to respond.” The authors go on to explain that these sorts of indicators do not reflect the underlying relationships between biophysical stocks and the specific value that they provide to humans via service provision. This is also a highly spatially-dependent issue, since the value of most ecosystem services depends on their distribution relative to the location of beneficiaries. In a certain sense, energy-climate modellers have been spoiled by the ideally global nature of carbon – one ton of CO2 emitted in London is atmospherically equivalent to one ton of CO2 emitted in Lucknow. Impacts to ecosystem services by energy systems, on the other hand, vary widely depending on local context.
Taking an overly narrow view of environmental outcomes is not only detrimental to ecosystems, it is also harmful to other important public policy considerations like human health. An example cited by Hooper et al (2018) of this problem was the UK government’s historical incentivisation of diesel vehicles to reduce carbon emissions, which resulted in increased air pollution and respiratory illness due to higher nitrogen dioxide emissions. If air quality effects were given adequate consideration, a different policy direction may have been chosen. Generally speaking, measures taken to improve the health of ecosystems work in tandem with improving human health.
While mostly absent in energy-climate pathways, there is a substantial body of literature on energy-ecosystem service interactions, which cover a variety of regulating (e.g. soil formation, air quality, erosion control) and provisioning (e.g. water availability, food, fiber production) services for different energy technologies. However, these studies rarely cover more than a few ecosystem services, making it difficult to account for comprehensive environmental impact. Further, many studies do not necessarily take into account the full life cycle impact of technologies, with upstream material extraction and related impacts (especially those that occur in other countries) often not included, further limiting the breadth of possible impacts to ecosystems. Lovett et al. (2015) is an example of an approach that succeeds in avoiding these shortcomings, as can be seen in the ecosystem services table below, which was used to evaluate the full life cycle impacts of UK-produced biomass crops. As biophysical data availability improves and more comprehensive frameworks for ecosystem service valuation are established, how will energy policy researcher adjust their methodologies to better holistically understand the ecological impacts of different energy pathways?
Level 1 | Level 2 description | Matrix columns | Level 3 description |
---|---|---|---|
Cultural | Intellectual representations of ecosystems (of environmental settings) | 27 | Non-use |
26 | Spiritual & symbolic | ||
Physical / experiential use of ecosystems (environmental setting) | 25 | Information & knowledge | |
24 | Non-extractive recreation | ||
Regulation & maintenance | Regulation of biotic environment | 23 | Pest & disease control (including invasive alien species) |
22 | Lifecycle maintenance, habitat & gene pool protection | ||
Regulation of physico-chemical environment | 21 | Noise regulation | |
20 | Pedogenesis & soil quality regulation | ||
19 | Water quality regulation | ||
18 | Atmospheric regulation | ||
Flow regulation | 17 | Mass flow regulation | |
16 | Water flow regulation | ||
15 | Air flow regulation | ||
Regulation of biophysical environment | 14 | Dilution, filtration & sequestration | |
13 | Bioremediation | ||
Provisioning | Energy | 12 | Renewable abiotic energy |
11 | Biomass-based energy | ||
Non-food biotic materials | 10 | Genetic materials | |
9 | Chemicals from plants & animals | ||
8 | Plant & animal fibres & structures | ||
Water supply | 7 | Water for industrial & energy uses | |
6 | Water for agricultural use | ||
5 | Water for human consumption | ||
Nutrition | 4 | Marine plants, algae & animals for food | |
3 | Freshwater plants & animals for food | ||
2 | Terrestrial plants & animals for food | ||
Supporting | 1 | Processes & functions (e.g. nutrient cycling, photosynthesis) |
UK low energy demand scenarios, BECCS, and afforestation
The concept of energy pathways in Integrated Assessment Models (IAMs) has become an essential way for energy researchers to simulate how different combinations and scales of energy technologies and underlying demand drivers can meet climate targets in the future (i.e. net-zero 2050 for countries in the Global North). Recent examples of energy pathways can be found in the IPCC’s Special Report for 1.5˚C and the recently release AR6 report. One of the major energy-climate challenges that CREDS has addressed through the Positive Low Energy Futures (PLEF) report is the inclusion of a broader set of demand-side measures into energy pathways for the UK. While prior low energy demand pathways constructed by Grubler et al. (2018) and Van Vuuren et al. (2018) contributed global scenarios, PLEF was the first-ever national low energy demand pathway. By adding more aggressive demand-side measures in bottom-up sectoral models that are normally overlooked in conventional energy pathways, the PLEF scenarios demonstrated how energy demand reduction in the UK can play a prominent role in simultaneously meeting climate targets, sustaining or improving human well-being, and reducing required energy infrastructure investments costs.
Since the purpose of the PLEF report was to explore the impact of energy demand changes on GHG emission trajectories in the UK, the report did not explicitly analyse impacts to ecosystem services related to the different scenarios. However, two major findings from the PLEF report have large potential ecological ramifications:
- A smaller energy system moderates the technical challenges of building out low-carbon infrastructure
- Lowering energy demand reduces reliance on high-risk engineered removals
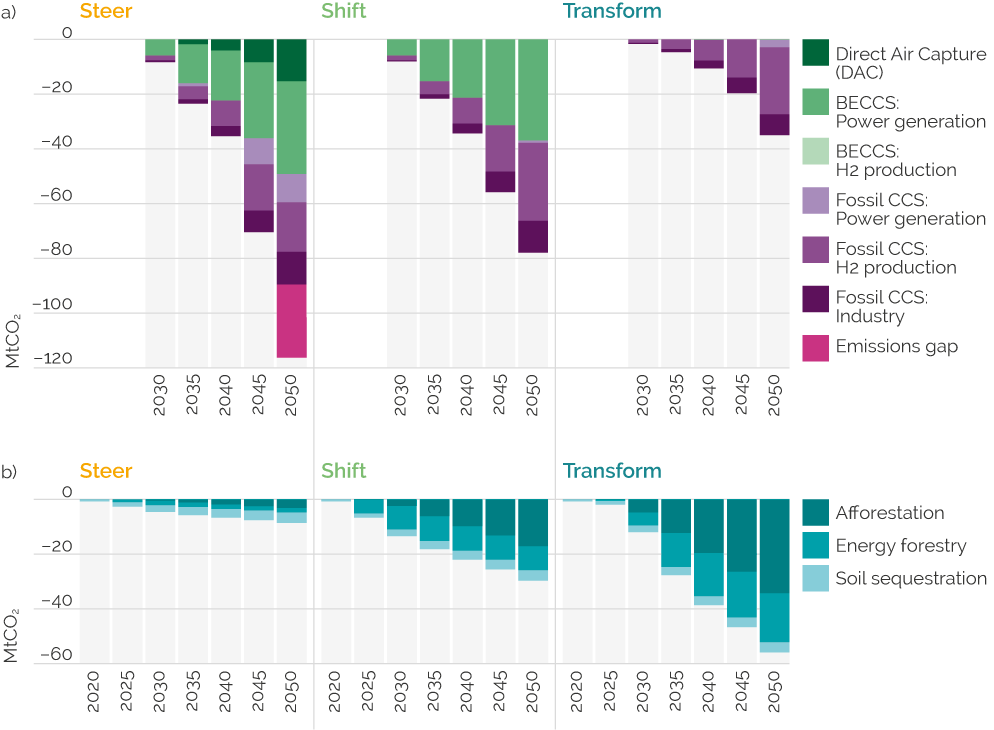
These conclusions are centred around the fact that without significant demand reduction in the UK, electricity production in a net-zero economy may need to be 2.5 times as much as current levels. Additionally, more carbon removal is required in current policy pathways (Steer Scenario), introducing a much higher need for Bioenergy with Carbon Capture and Storage (BECCS). The figure above shows how CO2 sequestration requirements differ in each of the PLEF scenarios (IAM assumptions in PLEF model: for BECCS, no limit was imposed for the Steer scenario, a 37 Mt CO2 limit was imposed for the Shift scenario, and no BECCS were permitted for the transform scenario. Inversely, more aggressive forestry replanting rates were permitted for the Transform and Shift scenarios relative to the Steer scenario).
In summary, conventional net-zero energy pathways are characterised by large deployments of BECCS and direct air capture, whereas low energy demand pathways feature more afforestation and/or reforestation, with less aggregate emissions needing to be offset. Additionally, since low energy demand pathways include dietary shifts away from meat consumption, there is less pressure on agricultural land. What do these takeaways mean for ecosystem service and biodiversity outcomes?
Potential implications for ecosystem services
While many of the same human activities that cause climate change also harm biodiversity, some interventions, like non-native monoculture plantations or renewable energy projects sited in fragile ecosystems, can mitigate climate change while harming biodiversity. This raises the possibility of a low-carbon but biodiversity-poor future. In order to avoid such a scenario, researchers have highlighted the risks of energy pathways that could endanger ecosystem services like food production and soil health, such as a high reliance on BECCS, pdf. At a macro-level, a reduced reliance on BECCS and increased focus on reforestation is understood to be positive for sustainability. This argument is aptly summarised in Chapter 5, pdf of the AR6 IPCC report on Demand, Services, and Social Aspects of Mitigation:
Increased demand for biomass can increase the pressure on forest and conservation areas (Cowie et al. 2013) and poses a heightened risk for biodiversity, livelihoods, and intertemporal carbon balances (Creutzig et al. 2021c; Lamb et al. 2016) requiring policy and regulations to ensure sustainable forest management which depends on forest type, region, management, climate, and ownership. This suggests that demand-side actions hold sustainability advantages over the intensive use of bioenergy and BECCS, but also enable land use for bioenergy by saving agricultural land for food.
The IPCC authors claim that low energy demand pathways have a clear advantage in the realm of general ecological sustainability. However, as Holland et al. (2016) have demonstrated, there is a risk of making speculative causal claims between high-level biophysical measures and ecosystem service provision and value:
Land use change can be used as a proxy for negative pressures on biodiversity (Santoyo-Castelazo and Azapagic, 2014), favouring energy pathways with smaller spatial footprints. Such analysis does not incorporate underpinning ecological factors such as patterns of species distribution in relation to environmental conditions.
While likely impractical at a global level, regional-scale analyses of the impacts of BECCS on ecosystem services have been conducted. In the literature, the most prominent study on this topic is Donnison et al (2020), who explored valuations of size- and site-specific BECCS deployments in the UK after calculating net welfare generated by changes to ecosystem services. The ecosystem values they modelled were agricultural output, soil organic carbon, natural flood management, water stress, and landscape availability. Out of the 5 sites that were modelled for 500 MW BECCS plants using local biomass resources, two returned high welfare gains, two generated low welfare gains, and one generated a welfare loss. When the plant sizes were increased to 1 GW each, all sites but one generated welfare losses, demonstrating the advantage of relatively smaller, distributed BECCS facilities over larger ones. However, without the economies of scale of large plants, the profitability of hypothetical small BECCS plants would be questionable. The authors concluded that “The feasibility of IAM scenarios should be assessed through their integration with spatially explicit environmental models… at a granular and site-specific level.” This suggests that while it is possible for BECCS to play a role in climate change mitigation and ecosystem service enhancement, energy-climate pathways that call on very high levels of BECCS deployment may have an overall negative impact on ecological integrity.
With regards to the potential for afforestation/reforestation efforts to generate welfare gains through improved ecosystem services, the literature is mixed but leans towards a range of possible positive outcomes under good management regimes. In the literature on reforestation and biodiversity, Paul et al (2016) provide a sound illustration of how to quantify the carbon-biodiversity trade-off through choice of tree species and planting width. This is also known as ‘planting the right trees in the right place.’ Their results showed that managing for carbon and biodiversity is possible and highly preferable, recommending that biodiversity assessments be fully integrated into carbon markets. The principle of good management regimes having an outsized impact on ecological outcomes also applies to bioenergy. A recent paper by the same team that conducted the UK BECCS study found that switching land from food production to non-food bioenergy crops, like willow and poplar, had positive outcomes for biodiversity.
In the Positive Low Energy Futures Report, neither BECCS nor afforestation are defined in a specific manner, making it difficult to say with any certainty what the outcomes for ecosystem services might be. However, we can infer that due to the limitations that govern the large scale expansion of BECCS, expanding right-fitting reforestation efforts to the scale called for in our report may be more ecologically preferred. Further, the reduction in raw material extraction resulting from a smaller energy system may in itself provide opportunities for improving natural capital stocks. At the end of the day, this analysis has demonstrated that spatially-explicit tools are still necessary to rigorously test out these hypotheses.
Moving forward
It would come as no surprise to environmental managers that the joint IPCC-IPBES report concluded that “measures narrowly focused on climate mitigation and adaptation can have direct and indirect negative impacts on nature and nature’s contributions to people.” Energy efficiency practitioners in particular are accustomed to framing their interventions within the context of co-benefits to human health and well-being. Similarly, the low energy demand community (myself included) have many hunches about how their scenarios can preserve and improve biospherical integrity.
Do low energy pathways provide a better chance of sustaining biodiversity and improving essential ecosystem services than conventional energy pathways? While it is tempting to conclude with an unwavering yes, in reality the answer is that it depends. For the two examples of climate mitigation methods provided – BECCS and afforestation – the net effects on ecosystem services depend on local context and management practices.
The ADVENT project (ADdressing Valuation of Energy and Nature Together), which is currently underway at the UK Energy Research Centre, has sought to take on this challenge by “quantify[ing] the implications of differing future UK low-carbon energy pathways for stocks of natural capital (e.g. groundwater and natural habitats) and for the provision of ecosystem services (e.g. irrigation, visual amenity, recreation).” In order to do this, the team has developed a modelling tool that “can be used to examine how that energy system might best be configured to minimise damage to ecosystem services.” While their final report is forthcoming, their research approach and tools provide invaluable direction to energy modellers seeking to integrate wider ecological outcomes into their analyses.
There are profound challenges with evaluating a broader suite of ecological impacts of our energy system, including data gaps, methodological challenges, and spatial heterogeneity. As a global leader in decarbonisation of energy systems, the UK has made laudable progress to meet its promise to reduce emissions by 78% from 1990 levels by 2035. However, legally binding targets also exist for other environmental outcomes, such as the Environment Act recently passed by Parliament. Integrating a comprehensive ecosystem services assessment framework into energy pathways is therefore a critical task for securing low-carbon and biodiversity-strong futures.
Dan Alberga is a summer research assistant at CREDS working on policy-related aspects of the Positive Low Energy Futures report. He is a Master’s student at the Yale School of the Environment specialising in energy policy. He is interested in demand-side interventions, energy services and human well-being, and energy-ecology interactions.
Banner photo credit: Steph Ferguson